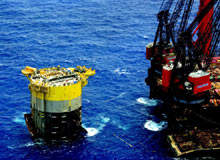
The present economic recession has resulted in a flattening of global energy demand but it is widely expected that this is temporary and that the long-term trend for increasing global energy demand will continue. To meet this increasing demand, all sources of energy and gains in energy efficiency will be needed.
As the ‘easy oil’ declines, the world will need to develop more difficult hydrocarbon sources including oil sands, heavy oil and oil shale as well conventional oil in harsh and ultra deepwater environments and the Arctic. This article addresses the technology challenges associated with future exploration and production of oil and gas in deepwater and the Arctic offshore and identifies and discusses technology pathways to enable these developments in a commercially feasible and sustainable manner.
High-level challenges
The global deepwater basins have already delivered substantial volumes of oil and gas and still hold significant promise. However the challenges of the future yet-to-find portfolio are expected to be considerable, including:
- increasing water depth up to 3,000m and beyond
- distances remote from existing infrastructure
- low reservoir pressure requiring artificial lift
- increased uncertainty due to imaging difficulties
- smaller recoverable volumes as basins mature.
It is widely anticipated that the Arctic holds significant volumes of oil and gas but exploration of the region is still in its early stages relative to other worldwide hydrocarbon producing basins. To date Arctic development and production has been in light to moderate ice conditions using islands or gravity base structures (GBS) in relatively shallow water or disconnectable floating systems. Challenges for the future include:
- Combination of water depth beyond the feasible range of GBS and moderate to heavy ice conditions
- Combination of sea ice and icebergs
- Hard, rock-strewn seafloors
- Emergency response
- Winterisation of facilities including design for fire and blast
- Footprint reduction.
The above challenges are explored further below with the objective of further identifying the technical challenges, development areas and pathways towards new and improved solutions.
How well do you really know your competitors?
Access the most comprehensive Company Profiles on the market, powered by GlobalData. Save hours of research. Gain competitive edge.
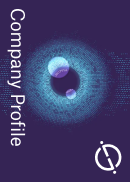
Thank you!
Your download email will arrive shortly
Not ready to buy yet? Download a free sample
We are confident about the unique quality of our Company Profiles. However, we want you to make the most beneficial decision for your business, so we offer a free sample that you can download by submitting the below form
By GlobalDataDeepwater harsh environments
Development systems in harsh environments already exist, for example Schiehalion, West of the Shetlands, and Kristin, offshore Norway. Harsh environment floaters have been shown to be successful, but not without technical difficulties including mooring line failures, some riser failures and hull cracking.
For weathervaning floating production, storage and offloading (FPSO), a key problem is the definition of appropriate environmental conditions for mooring design, in particular the magnitude and direction of waves, winds and currents in relation to the vessel heading.
Specific technology development areas needed for floaters in a harsh environment in deeper waters include:
Improved and validated design tools for response-based design conditions for weathervaning FPSOs. Key responses of interest are maximum offset for mooring design, maximum roll and pitch, maximum topside accelerations.
Better approaches for modelling wave and green water impact on the deck structure.
Ultradeep floaters with direct vertical access
Ultradeepwater is typically more than 1,500m. Prospects in ultradeepwater are seen in the Gulf of Mexico, Brazil and West Africa.
Some of these prospects, including BS4 in Brazil, are associated with heavy oil. Others, such as the Perdido spar development in Gulf of Mexico, in a water depth of 2,500m, are low-pressure reservoirs and require artificial lift.
When a reservoir can be accessed and drained from a single drilling centre, use of a production system with direct vertical access (DVA) is advantageous because it can be used to drill and complete the wells at a considerably lower cost than using deepwater drilling rigs, which are in high demand. Systems that may provide DVA are typically the spar and deep draft semi (for mild environments such as West Africa or South China Sea).
A key constraint in extending the spar to deeper water is the increasing difficulty of accommodating many risers. As the riser weight increases, the size of buoyancy ‘aircans’ grows and this affects the size of the hull.
A series of innovations implemented in the Shell Perdido spar development deal effectively with the riser issue and resolve the matter of low reservoir pressure. In these innovations flow from several wells is channelled into a caisson separator at the base of the spar, which separates liquids from gas.
Liquids are pumped separately up the riser using an electric submersible pump (ESP), thereby providing the required lift. This way, flow from 25 wells requires only five production risers, considerably reducing the size of the moonpool and hence the size of the spar.
The Perdido spar also incorporates a drilling riser, which is used to drill and complete the wells as subsea wells. Use of DVA for drilling reduces costs considerably and provides well intervention capability over the producing life.
The above innovations provide an effective holistic (complete system) solution to the twin challenges of ultradeep water and low-pressure reservoirs. It also leads to lower drilling costs.
It is anticipated that maturing and extension of these technologies will be an enabler for many other prospects.
Potential of deep draft semi
The motion characteristics of a conventional semi can be improved by increasing the draft by 10-15m and retaining many of the advantages of the semi shape, notably nearshore integration with the topsides. The improved motion characteristics can enable the use of steel catenary risers in the Gulf of Mexico and other areas.
The improved motion may also enable the semi to be used as a DVA host system in relatively mild environments, such as the South China Sea or West Africa. Nevertheless, top-tensioned risers will need to accommodate large stroke.
Concepts for large stroke tensioners have been developed but require additional testing and qualification to bring these concepts to ‘project ready’ status. Additionally the stiffness of the riser tensioners influences the platform motions and an integrated solution for hull, moorings and risers is required.
Subsea production systems
A clear trend in the offshore industry is that increasing amounts of the production equipments are installed on the seabed. Subsea-to-beach solutions, which eliminate the need for a floating platform, have been implemented in the flagship developments of Ormen Lange and Snovit offshore Norway.
These are gas developments, where it is easier to establish reliable flow assurance strategies for long distances. A next logical step, relevant to gas developments, is subsea compression, generally needed later in field life.
For Ormen Lange future compression is required. A pilot subsea compression is being constructed and, if successful, may be implemented, thereby obviating the need for floater compression.
Challenges that need to be resolved to enable subsea compression include the need for intervention for maintenance or replacement of components, system reliability, power supply and power conversion.
For oil developments the use of subsea separation, as illustrated earlier for the Perdido development project, enables pumping of the liquids to provide artificial lift. These techniques can be matured further to enable pumping over longer distances to enable subsea-to-beach developments for oil fields.
Arctic challenges and technology development areas
Challenges for oil and gas facilities in the arctic fall into two categories. The first, and conventional, focus of Arctic technology is overcoming the physical environment.
The second is doing this in a manner that meets regulatory requirements and increasing societal expectations related to environmental performance and social benefits.
With respect to the first of these challenges, significant progress has been made through the development of ISO Draft International Standard 19906, addressing design, construction, transportation, installation, and removal of offshore structures, related to the activities of the petroleum and natural gas industries, in Arctic and cold regions. Focus areas for advancement and extension of Arctic development include:
- Safety critical systems: operation of manned installations in cold temperatures requires winterisation of facilities to maintain temperatures above freezing, addressing fire and blast, spray icing and ice accretion, and emergency response, including platform escape, evacuation, and rescue under very difficult conditions. Continuous advancement is needed to increase safety, bring down costs, and increase capability to handle more difficult environmental conditions.
- Ice and iceberg data: long-term and reliable databases are needed to predict ULS (ultimate limit state) and rare ALS (accidental limit state) design conditions. Refinement and extension of existing databases are needed to reduce conservatism and support entry into new areas. Furthermore, recent changes in summer and winter ice cover in the Arctic call into question the reliability of using the past as a predictor of the future. Warming could also lead to an increase in iceberg calving affecting the frequency and size distributions for iceberg-prone areas.
- Ice actions and structure response: specific issues include reducing the conservatism contained in ISO 19906 and better predicting structure response, particularly for lighter structures susceptible to ice induced vibration. Finally, addressing icebergs in a sea ice matrix, as found offshore Greenland, poses a new and unmet challenge to be overcome.
- Deepwater and severe ice conditions: designing for the combination of deepwater – defined as water depth beyond the feasible water depth for gravity base structures – and severe ice conditions is a key technical challenge. Subsea production has potential, given the right reservoir conditions and sufficient open water season for drilling, inspection, maintenance and repair operations. But challenges remain, including flow assurance, protection from deep keeled ice pressure ridges and icebergs, and availability, due to inaccessibility during the ice bound season.
Reducing footprint
Reduced footprint is becoming a significant driver from the standpoint of regulatory requirements and societal expectations. Footprint in its broadest sense includes physical dimensions, air emissions, liquid and solid discharge, and sound emissions.
While many of these areas fall outside the scope of Arctic technology, Arctic success will require integrated solutions addressing these elements.
Reduced footprint also creates a unique driver for innovation and development and deployment of new technology. Ongoing technology efforts within Shell are aimed at adapting external solutions, such as from the pile driving industry to reduce sound transmission from fixed installations, and investigation of technology from across upstream and downstream that can be brought to bear.
Conclusion
In the new era characterised by increased demand and less easy oil, the deepwater and Arctic offshore represent opportunities to contribute significantly to existing supply. Some of the key technical challenges facing future development of oil and gas prospects in these regions have been provided and technology areas highlighted.
For deepwater, specific technology pathways have been highlighted using new, holistic or complete system solutions including wet tree DVA with subsea separation and pumping for low-pressure reservoirs, and subsea to beach plus subsea compression for gas fields.
For the Arctic specific technology pathways include continued advancement and extension of solutions to overcome the physical environment including improved definition of the environment (and possible future changes), substructure response to ice induced vibration, and development of systems beyond the economic limit of gravity base structures. These pathways must also consider means of reducing footprint to meet stakeholder expectations.
Shell favours a dual strategy of pathways that address specific technology challenges and holistic integrated concepts, striving for solutions that deliver commercial success in a sustainable and responsible manner.